
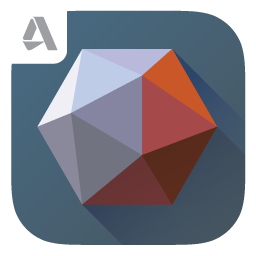
To achieve this, a single representative image, chosen subjectively from the image stack, is analyzed with the region-of-interest (ROI) function, which allows each individual pixel's brightness to be measured. Simply speaking, the grayscale is reduced to black and white, representing absence or presence of tissue. Following import of the volumes in Osirix, a process called thresholding determines the level of grayscale value above which a pixel is considered to be tissue.
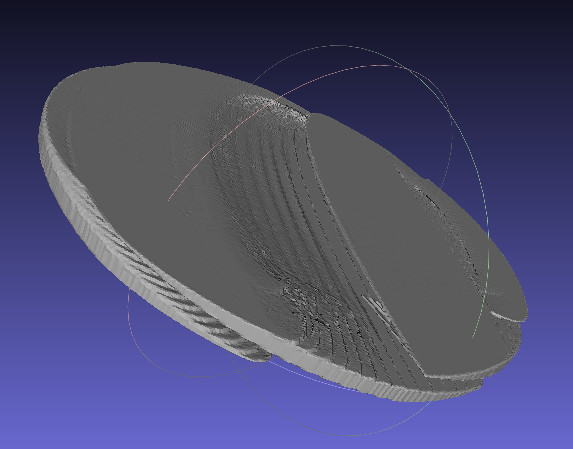
#Osirix dicom to stl free#
Then, the image stacks are converted into a printable format, STL, using a popular free DICOM viewer, Osirix Lite (Version 5.9, Pixmeo Sarl, Bernex, Switzerland). First, the volumes are exported in DICOM format.
#Osirix dicom to stl manual#
The individual steps required for manual conversion in order to prepare print-ready STL files are shown in Figure 2. Neither volumes in the proprietary format of each machine nor DICOM files can be used directly for 3D printing. For STIC acquisitions, single volumes (diastole or systole, assessed subjectively) were selected. All other volumes, including spatiotemporal image correlation (STIC) volumes of the fetal heart, had been acquired with a transabdominal probe. All embryonic volumes (up to 10 weeks) and some fetal volumes (between 11 and 14 weeks) had been acquired transvaginally. Volumes included several embryos and fetuses ranging from 14 to 74 mm in crown–rump length, two mid-trimester fetal faces and several normal and abnormal fetal hearts at 19–29 weeks. Mechanical sweep curved-array transabdominal or transvaginal transducers had been used (Voluson E8 machine, equipped with RAB6D and RIC6-12 transducers, GE Medical Systems, Zipf, Austria iU22 machine, equipped with 3D9-3v transducer, Philips Medical Systems, Bothell, WA, USA). They had been acquired using diagnostic US systems capable of 3D imaging and of exporting volumes as image stacks, initially in DICOM (Digital Imaging and Communications in Medicine) format, a standard for medical image storage, and later directly, in STL (stereolithography) format. From volume to DICOM to printable STLįetal US volumes that had been acquired as part of routine diagnostic workflow and with known patient outcomes were selected subjectively for clarity and resolution. This Editorial describes how new, freely available tools for 3D printing can be used for volumes obtained with current US systems, and gives detailed protocols for this conversion using both standard US systems, which are not capable of 3D export of volumes, and newer systems, which offer export of volumes in a printable format. However, 3D printing from fetal ultrasound data has been limited by the fact that it entails labor-intensive post-processing, including slice-by-slice manual segmentation and reassembly. Advances in consumer electronics and the availability of 3D printing services have now made 3D printing an affordable reality. 3D printing using volume US data taken from commercially available US systems was reported only very recently 9, 10. Combinations of prenatal volume data from US imaging and magnetic resonance imaging (MRI) have also been used 6- 8. 5, who used two custom-designed scanners, one of which was used for motion gating. Printing of 3D heart models from fetal US data was pioneered in 2000 by Deng et al. In obstetrics and gynecology, it is now practically synonymous with either cross-sectional reformatting or surface rendering, both displayed on a computer screen. 3D-US has improved considerably the understanding of normal and abnormal sonographic anatomy 4. More than a century later, 3D analysis and rendering of ultrasound (US) volumes has become routine due to the wide availability of 3D-capable US systems. (Image modified from O'Rahilly and Müller 3. For comparison with Figures 2 and 3, this enlarged physical plaster plate model was generated by O'Rahilly and Müller, according to the ‘Plattenmodellirmethode’ of Born 2, from sagittal histological sections of an embryo at Carnegie stage 21, with crown–rump length 24 mm.
